تاريخ الفيزياء
علماء الفيزياء
الفيزياء الكلاسيكية
الميكانيك
الديناميكا الحرارية
الكهربائية والمغناطيسية
الكهربائية
المغناطيسية
الكهرومغناطيسية
علم البصريات
تاريخ علم البصريات
الضوء
مواضيع عامة في علم البصريات
الصوت
الفيزياء الحديثة
النظرية النسبية
النظرية النسبية الخاصة
النظرية النسبية العامة
مواضيع عامة في النظرية النسبية
ميكانيكا الكم
الفيزياء الذرية
الفيزياء الجزيئية
الفيزياء النووية
مواضيع عامة في الفيزياء النووية
النشاط الاشعاعي
فيزياء الحالة الصلبة
الموصلات
أشباه الموصلات
العوازل
مواضيع عامة في الفيزياء الصلبة
فيزياء الجوامد
الليزر
أنواع الليزر
بعض تطبيقات الليزر
مواضيع عامة في الليزر
علم الفلك
تاريخ وعلماء علم الفلك
الثقوب السوداء
المجموعة الشمسية
الشمس
كوكب عطارد
كوكب الزهرة
كوكب الأرض
كوكب المريخ
كوكب المشتري
كوكب زحل
كوكب أورانوس
كوكب نبتون
كوكب بلوتو
القمر
كواكب ومواضيع اخرى
مواضيع عامة في علم الفلك
النجوم
البلازما
الألكترونيات
خواص المادة
الطاقة البديلة
الطاقة الشمسية
مواضيع عامة في الطاقة البديلة
المد والجزر
فيزياء الجسيمات
الفيزياء والعلوم الأخرى
الفيزياء الكيميائية
الفيزياء الرياضية
الفيزياء الحيوية
الفيزياء العامة
مواضيع عامة في الفيزياء
تجارب فيزيائية
مصطلحات وتعاريف فيزيائية
وحدات القياس الفيزيائية
طرائف الفيزياء
مواضيع اخرى
Constrained evolutionary structure of Einstein’s equations
المؤلف:
Heino Falcke and Friedrich W Hehl
المصدر:
THE GALACTIC BLACK HOLE Lectures on General Relativity and Astrophysics
الجزء والصفحة:
p 183
29-1-2017
1895
Constrained evolutionary structure of Einstein’s equations
In GR the basic field is the spacetime metric gμν, which comprises the gravitational and inertial properties of spacetime. It defines what inertial motion is, namely a geodesic
(1.1)
with respect to the Levi-Civita connection
(1.2)
(Since inertial motion is ‘force free’ by definition, you may rightly ask whether it is correct to call gravity a ‘force’.) The gravitational field gμν is linked to the matter content of spacetime, represented in the form of the energy–momentum tensor Tμν, by Einstein's equations
(1.3)
Due to the gauge invariance with respect to general differentiable point transformations (i.e. diffeomorphisms) of spacetime, one has the identities (as a consequence of Noether's second theorem)
(1.4)
Being ‘identities’ they hold for any Gμν, independent of any field equation. With respect to some coordinate system xμ = (x0, . . . x3) we can expand (1.4) in terms of ordinary derivatives. Preferring the coordinate x0, this reads:
(1.5)
Since Gμν contains no higher derivatives of gμν than the second, the right hand side of this equation also contains only second x0 derivatives. Hence (1.5) implies that the four components G0ν only involve first x0-derivatives. Now choose x0 the as time coordinate. The four (0, ν)-components of (1.3) then do not involve second time derivatives, unlike the space–space components (i, j). Hence the time-time and time–space components are constraints, that is, equations that constrain the allowed choices of initial data, rather than evolving them.
This is not an unfamiliar situation as it similarly occurs for Maxwell's equations in electromagnetism (EM). Let us recall this analogy. We consider the four dimensional form of Maxwell's equations in terms of the vector potential Aμ, whose antisymmetric derivative is the field tensor Fμν := ∂μAν − ∂ν Aμ, comprising the electric (Ei = F0i ) and magnetic (Bi = −Fjk, i jk cyclic) fields. Maxwell's equations are
(1.6)
Due to its antisymmetry, the field tensor obviously obeys the identity
(1.7)
which here is the analogue of (1.4), an identity involving third derivatives in the field variables. Using (1.7) in the divergence of (1.6) yields
(1.8)
which shows that Maxwell's equations imply charge conservation as the integrability condition. Let us interpret the role of charge conservation in the initial-value problem. Decomposing (1.7) into space and time derivatives gives
(1.9)
Again the right hand side involves only second time derivatives implying that the zero-component of (1.6) involves no second time derivatives. Hence the time component of (1.6) is merely a constraint on the initial data; clearly it is just Gauss's law ∇ · E − 4πρ = 0. Its change under time evolution according to Maxwell's equations is
(1.10)
where we have used the identity (1.8) in the second step. Suppose now that on the initial surface of constant x0 we put an electromagnetic field which satisfies the constraint, E0 = 0, and which we evolve according to Ek = 0 (implying ∂k Ek = 0 on that initial surface). Then (1.10) shows that charge conservation is a necessary and sufficient condition for the evolution to preserve the constraint.
Let us return to GR now, where the overall situation is entirely analogous. Now we have four constraints
(1.11)
and six evolution equations, which we write
(1.12)
The identity (1.4) now implies
(1.13)
which parallels (1.8). Here, too, the time derivative of the constraints is easily calculated,
(1.14)
by using (1.13) in the last step. Now consider again the evolution of initial data from a surface of constant x0. If they initially satisfy the constraints and are evolved via Ei j = 0 (hence all spatial derivatives of Eμν vanish initially) they continue to satisfy the constraints if and only if the energy momentum tensor of the matter satisfies
(1.15)
Hence we see that the ‘covariant conservation’ of energy–momentum, expressed by (1.15), plays the same role in GR as charge conservation plays in EM. This means that you cannot just prescribe the motion of matter and then use Einstein's equations to calculate the gravitational field produced by that source. You have to move the matter in such a way that it satisfies (1.15). But note that at this point there is a crucial mathematical difference to charge conservation in EM: charge conservation is a condition on the source only, it does not involve the electromagnetic field. This means that you know a priori what to do in order not to violate charge conservation. However, (1.15) involves the source and the gravitational field. The latter enters through the covariant derivatives which involve the metric gμν through the connection coefficients (1.2). Hence here (1.15) cannot be solved a priori by suitably restricting the motion of the source. Rather we have a consistency condition which mutually links the problem of motion for the sources and the problem of field determination. It is this difference which makes the problem of motion in GR exceedingly difficult. (A brief and lucid presentation of this problem, drawing attention to its relevance in calculating the generation of gravitational radiation by self-gravitating systems. A broader summary, including modern developments.)
For example, for pressureless dust represented by T μν = ρc2 UμUν, where ρ is the local rest-mass density and Uμ is the vector field of four-velocities of the continuously dispersed individual dust grains, (1.15) is equivalent to the two equations:
(1.16)
(1.17)
The first states the conservation of rest-mass. The second is equivalent to the statement that the vector field Uμ is geodesic, which means that its integral lines (the world lines of the dust grains) are geodesic curves (1.1) with respect to the metric gμν. Hence we see that in this case the motion of matter is fully determined by (1.15), i.e. by Einstein's equations, which imply (1.15) as integrability condition. This clearly demonstrates how the problem of motion is inseparably linked with the problem of field determination and that these problems can only be solved simultaneously. The methods used today use clever approximation schemes. For example, one can make use of the fact that there is a difference of one power in κ between the field equations and their integrability condition. Hence, in an approximation in κ, it is consistent for the nth order approximation of the field equations to have the integrability conditions (equations of motions) satisfied to n - 1st order.
Clearly the problem just discussed does not arise for the matter-free Einstein equations for which Tμν ≡ 0. Now recall that black holes are described by matter free equations, too. Hence, the mathematical problem just described does not occur in the discussion of their dynamics. In this aspect the discussion of black hole scattering is considerably easier than, e.g., that of neutron stars.
الاكثر قراءة في الثقوب السوداء
اخر الاخبار
اخبار العتبة العباسية المقدسة
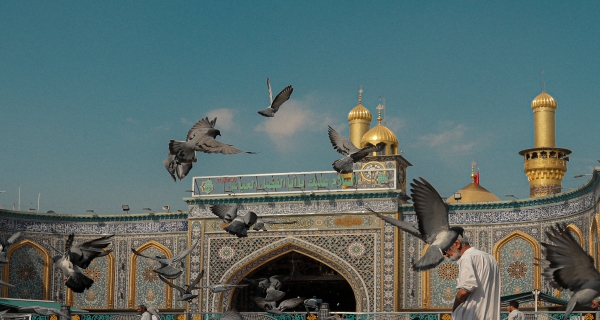
الآخبار الصحية
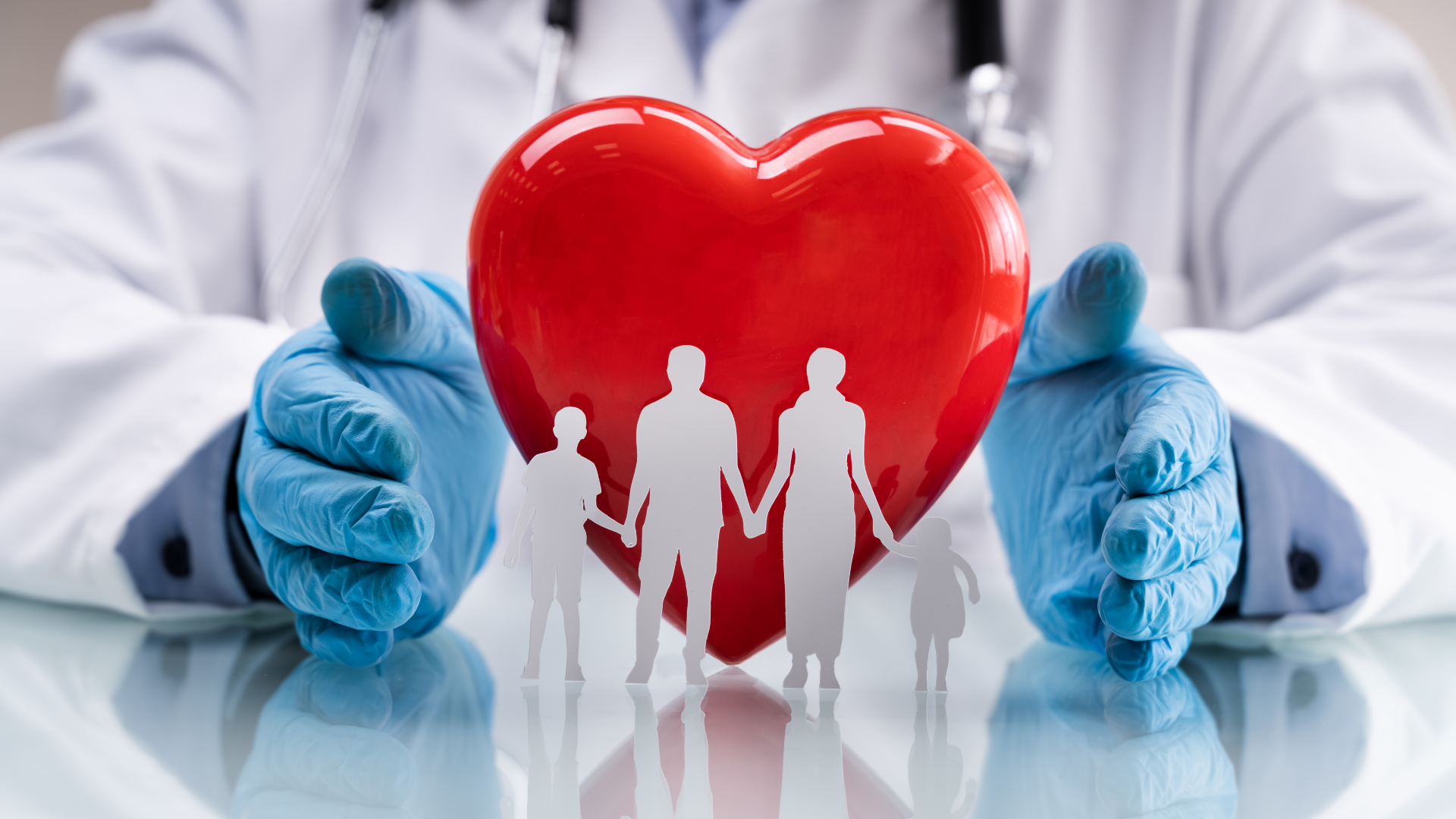